CUT&Tag : A Major Advancement in Chromatin Profiling
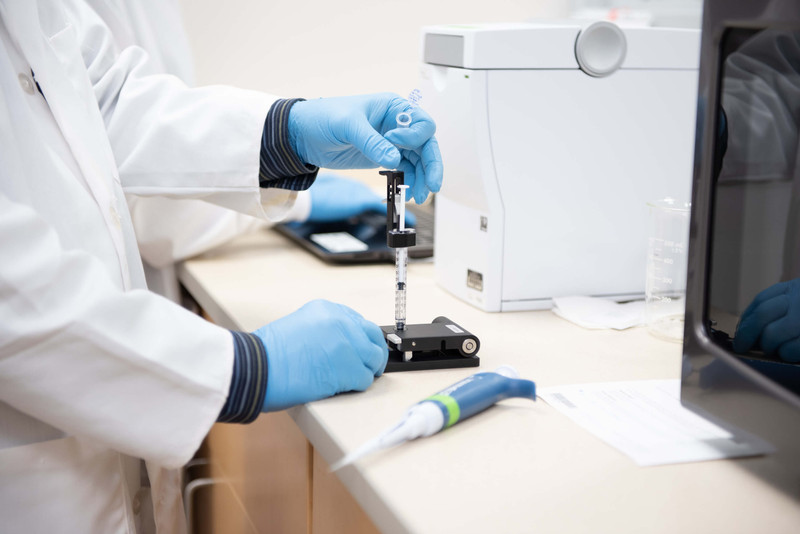
Since its development over three decades ago, Chromatin ImmunoPrecipitation (ChIP) has become the most widely used chromatin mapping method. However, ChIP suffers from a number of drawbacks that limit its accuracy and precision, thus restricting its utility toward the development of novel diagnostics or therapeutic tools1. Innovative chromatin mapping strategies, such as CUT&RUN2-5 and CUT&Tag6, overcome many of the limitations associated with ChIP and provide a means to launch a new era of chromatin research.
CUT&RUN and CUT&Tag : Modern Chromatin Mapping Methods
Cleavage Under Targets & Release Under Nuclease (CUT&RUN) is a novel chromatin mapping method that is faster, more accessible, and provides higher quality data compared to ChIP-based approaches. The development of ChIC (Chromatin ImmunoCleavage)5 / CUT&RUN2-4 is discussed more at length here. These approaches utilize a fusion of proteins A and G with micrococcal nuclease (pAG-MNase) to selectively cleave DNA fragments at genomic sites that have been tagged by an antibody specific to a protein or histone post-translational modification (PTM) of interest (Figure 1). Importantly, only the DNA interacting with the antibody-tagged protein / PTM of interest is extracted from solution, which greatly increases the signal-to-noise ratio while also enabling lower cell inputs and reduced sequencing depth requirements3, 4.
Cleavage Under Targets and Tagmentation (CUT&Tag), developed by Dr. Steven Henikoff’s group, is based on the same immunotethering principles5, 6. However, the CUT&Tag method fuses proteins A/G to a hyperactive Tn5 transposase (pAG-Tn5), instead of MNase. The pAG-Tn5 is then loaded with sequencing adaptors. When incubated with cells (or nuclei), the addition of magnesium activates pAG-Tn5 mediated tagmentation, in which the target sequence, bound by an antibody, is simultaneously fragmented and tagged with the sequencing adapters. The purified target DNA can be directly amplified and sequenced, eliminating tedious library preparation steps. As a result, CUT&Tag is more time efficient than CUT&RUN and amenable to even lower inputs, including single cells6. A detailed step-by-step comparison of the two techniques is provided in Figure 2 below.
Both CUT&RUN and CUT&Tag provide reliable data from low cell numbers, with rapid turnaround times through a streamlined workflow. Quality signal can be achieved with remarkably low read depth, so sequencing can be performed in-house on benchtop sequencers. These methods can also be scaled up and automated for high-throughput analysis7.
Improving Reliability and Reproducibility for CUT&RUN and CUT&Tag
CUT&RUN and CUT&Tag are both incredibly promising approaches for chromatin analyses. In fact, CUT&RUN has already been utilized with great success in >40 articles since its original publication in 2017. Additional technical developments and validated reagents will further extend the utility of these methods and improve the quality and precision of generated data.
Crucially, the inclusion of spike-in controls will allow normalization both within and across studies. The latest CUT&RUN protocol suggests using carryover E. coli DNA, introduced by the MNase fusion protein, for calibration3. Alternatively, addition of exogenous DNA spike-ins (e.g. E.coli, yeast) can also help normalize CUT&RUN data, particularly when using pAG-MNase with little to no carryover DNA (as is the case for EpiCypher’s pAG-MNase). However, while this may provide a control for library preparation, it will not account for sources of variability introduced earlier in the experiment, such as during antibody incubation or washing steps. Spike-in controls, introduced at the earliest experimental step, would account for these differences and provide more reliable data8.
Spike-in controls should also ideally be designed to allow in-application antibody validation. Similar to ChIP-seq, CUT&RUN and CUT&Tag rely on specific, high affinity antibodies to produce accurate data. However, as EpiCypher and others have demonstrated, many antibodies to histone PTMs display significant cross-reactivity to related PTMs9-14. We have addressed this deficit by developing SNAP-ChIP® spike-in technology, which uses DNA-barcoded recombinant nucleosomes for in-application antibody validation and data normalization in ChIP-seq experiments. EpiCypher provides a collection of highly specific and lot-verified SNAP-ChIP® Certified Antibodies, and has also begun testing these antibodies for compatibility in CUT&RUN.
To directly enable antibody assessment of antibody specificity in CUT&RUN / CUT&Tag, EpiCypher is developing our nucleosome-based spike-ins for compatibility with these approaches. Watch this space.
Shop CUTANATM CUT&Tag Products!
When to Tag and when to RUN
Both CUT&Tag and CUT&RUN are powerful methods that offer significant advantages over ChIP. However, researchers interested in chromatin mapping may be wondering which method to choose. Although the efficiency and advantages of CUT&Tag vs. CUT&RUN have not been extensively studied, comparison of these methods and early publications offer some clues:
• CUT&Tag offers a notable benefit over CUT&RUN in the elimination of library preparation steps, which significantly reduces time, resources, and cell input requirements.
• However, CUT&RUN outperforms CUT&Tag in profiling transcription factor binding, and can map at higher resolution due to the smaller size of the MNase protein and nature of its reaction3, 4, 6.
Thus, researchers should strongly consider their target type, resolution needs, input, and resource availability for library preparation when deciding between CUT&RUN and CUT&Tag. For example, a researcher interested in transcription factor binding would likely choose CUT&RUN, while another studying a histone PTM in a limited sample input would choose CUT&Tag.
Additionally, CUT&RUN has been widely demonstrated to yield robust results15-19, due in part to the availability of highly optimized protocols2, 3, 20. These altered CUT&RUN methods include modifications for reduced cell inputs. EpiCypher’s CUTANATM CUT&RUN protocol has been used to profile a few as 5,000 cells, but other labs are pushing the boundaries even further, down to the single cell level3, 21. Click here to learn more about CUTANA CUT&RUN assays and related products.
In contrast, CUT&Tag protocol development is still being worked out for various targets and / or sample types (download the most up to date version of EpiCypher's CUT&Tag protocol). Therefore, the best strategy for a researcher embarking on a new project would be to start with CUT&RUN to learn the immunotethering approach and test compatibility in their model system of interest. Once optimized, the researcher can then directly compare the robustness of CUT&RUN with CUT&Tag, particularly when it is desirable to perform studies using very low cell numbers or to circumvent library preparation.
Unsure how to proceed for your next study? Reach out to our technical support team and we will help you decide whether to “Tag” or “RUN” for your next experiment.
Uncharted Territory : Future Directions for Applications of CUT&RUN and CUT&Tag
Altered epigenetic mechanisms have been tied to numerous diseases, notably cancer22, diabetes23, and autoimmunity24. The field of clinical epigenetics is rapidly expanding, and multiple therapeutics with epigenetic targets have made their way through clinical trials to the pharmaceutical market. Histone PTMs and chromatin-associated proteins are among the most promising targets for epigenetic drugs (e.g. histone deacetylase [HDAC] inhibitors)25, highlighting the potential of CUT&RUN and CUT&Tag to facilitate significant advancements in the study of both disease and therapeutics.
The ease and affordability of CUT&RUN and CUT&Tag will increase general access to epigenetics research, creating more opportunities for discoveries with clinical relevance. For established epigenetics disease mechanisms, profiling can be completed with faster turnaround for improved diagnostics and monitoring on a clinical timescale. Likewise, these methods will also directly enhance drug development and response monitoring.
CUT&Tag is particularly notable in that it is amenable to single cell inputs6. Single cell sequencing and transcriptomics have revolutionized our ability to profile heterogeneous tissues and cell populations, such as tumors and immune cell infiltrates. This high-resolution analysis allows the characterization of rare cell populations and cell-to-cell variation that would otherwise be missed in bulk analysis26. Epigenetic profiling is the next frontier in single cell methods, facilitating analysis of chromatin features within heterogeneous samples27.
While many epigenetic techniques do not translate well to single cell applications, CUT&Tag provides a highly tractable method for single cell profiling of histone PTMs and other chromatin-associated proteins. This is especially relevant in the context of drug development and personalized medicine, enabling high-resolution characterization of diseased tissues, including identification of rare cell populations (e.g. drug-resistant cells) that may be highly important in determining prognosis and/or suitability of specific therapeutics28. Notably, the overall success of epigenetic drugs has been limited and highly variable across patients25. Single cell analysis might provide the information needed to target specific therapies to the patients most likely to respond positively.
Further advances in the applications of CUT&RUN and CUT&Tag may come through integration with other methods, such as RNA-Seq (i.e. multi-omics). Systems-level analysis performed in the same cells could provide unparalleled insight into the direct relationship between histone PTMs / chromatin-associated proteins and gene expression. Simultaneous profiling of the chromatin accessibility, transcriptome, and methylome from single cells has been previously demonstrated29, 30, providing a precedent for the feasibility of multi-omics using immunotethering techniques.
References
1. Park PJ. ChIP-seq: advantages and challenges of a maturing technology. Nat Rev Genet, 2009. 10(10): p. 669-80. (PubMed PMID: 19736561)
2. Meers MP, et al. Improved CUT&RUN chromatin profiling tools. Elife, 2019. 8: p. (PubMed PMID: 31232687)
3. Skene PJ, et al. Targeted in situ genome-wide profiling with high efficiency for low cell numbers. Nat Protoc, 2018. 13(5): p. 1006-19. (PubMed PMID: 29651053)
4. Skene PJ, Henikoff S. An efficient targeted nuclease strategy for high-resolution mapping of DNA binding sites. Elife, 2017. 6: p. (PubMed PMID: 28079019)
5. Schmid M, et al. ChIC and ChEC; genomic mapping of chromatin proteins. Mol Cell, 2004. 16(1): p. 147-57. (PubMed PMID: 15469830)
6. Kaya-Okur HS, et al. CUT&Tag for efficient epigenomic profiling of small samples and single cells. Nat Commun, 2019. 10(1): p. 1930. (PubMed PMID: 31036827)
7. Janssens DH, et al. Automated in situ chromatin profiling efficiently resolves cell types and gene regulatory programs. Epigenetics Chromatin, 2018. 11(1): p. 74. (PubMed PMID: 30577869)
8. Chen K, et al. The Overlooked Fact: Fundamental Need for Spike-In Control for Virtually All Genome-Wide Analyses. Mol Cell Biol, 2015. 36(5): p. 662-7. (PubMed PMID: 26711261)
9. Shah RN, et al. Examining the Roles of H3K4 Methylation States with Systematically Characterized Antibodies. Mol Cell, 2018. 72(1): p. 162-77 e7. (PubMed PMID: 30244833)
10. Rothbart SB, et al. An Interactive Database for the Assessment of Histone Antibody Specificity. Mol Cell, 2015. 59(3): p. 502-11. (PubMed PMID: 26212453)
11. Rothbart SB, et al. Poly-acetylated chromatin signatures are preferred epitopes for site-specific histone H4 acetyl antibodies. Sci Rep, 2012. 2: p. 489. (PubMed PMID: 22761995)
12. Fuchs SM, Strahl BD. Antibody recognition of histone post-translational modifications: emerging issues and future prospects. Epigenomics, 2011. 3(3): p. 247-9. (PubMed PMID: 22122332)
13. Nishikori S, et al. Broad ranges of affinity and specificity of anti-histone antibodies revealed by a quantitative peptide immunoprecipitation assay. J Mol Biol, 2012. 424(5): p. 391-9. (PubMed PMID: 23041298)
14. Fuchs SM, et al. Influence of combinatorial histone modifications on antibody and effector protein recognition. Curr Biol, 2011. 21(1): p. 53-8. (PubMed PMID: 21167713)
15. Liu N, et al. Direct Promoter Repression by BCL11A Controls the Fetal to Adult Hemoglobin Switch. Cell, 2018. 173(2): p. 430-42 e17. (PubMed PMID: 29606353)
16. Zheng XY, Gehring M. Low-input chromatin profiling in Arabidopsis endosperm using CUT&RUN. Plant Reprod, 2019. 32(1): p. 63-75. (PubMed PMID: 30719569)
17. Rothstein M, Simoes-Costa M. Heterodimerization of TFAP2 pioneer factors drives epigenomic remodeling during neural crest specification. Genome Res, 2020. 30(1): p. 35-48. (PubMed PMID: 31848212)
18. Federation AJ, et al. Highly Parallel Quantification and Compartment Localization of Transcription Factors and Nuclear Proteins. Cell Rep, 2020. 30(8): p. 2463-71 e5. (PubMed PMID: 32101728)
19. Chen Z, et al. Allelic H3K27me3 to allelic DNA methylation switch maintains noncanonical imprinting in extraembryonic cells. Sci Adv, 2019. 5(12): p. eaay7246. (PubMed PMID: 32064321)
20. Hainer SJ, Fazzio TG. High-Resolution Chromatin Profiling Using CUT&RUN. Curr Protoc Mol Biol, 2019. 126(1): p. e85. (PubMed PMID: 30688406)
21. Hainer SJ, et al. Profiling of Pluripotency Factors in Single Cells and Early Embryos. Cell, 2019. 177(5): p. 1319-29 e11. (PubMed PMID: 30955888)
22. Dawson MA, Kouzarides T. Cancer epigenetics: from mechanism to therapy. Cell, 2012. 150(1): p. 12-27. (PubMed PMID: 22770212)
23. Ling C, Ronn T. Epigenetics in Human Obesity and Type 2 Diabetes. Cell Metab, 2019. 29(5): p. 1028-44. (PubMed PMID: 30982733)
24. Mazzone R, et al. The emerging role of epigenetics in human autoimmune disorders. Clin Epigenetics, 2019. 11(1): p. 34. (PubMed PMID: 30808407)
25. McClure JJ, et al. Advances and Challenges of HDAC Inhibitors in Cancer Therapeutics. Adv Cancer Res, 2018. 138: p. 183-211. (PubMed PMID: 29551127)
26. Hwang B, et al. Single-cell RNA sequencing technologies and bioinformatics pipelines. Exp Mol Med, 2018. 50(8): p. 96. (PubMed PMID: 30089861)
27. Clark SJ, et al. Single-cell epigenomics: powerful new methods for understanding gene regulation and cell identity. Genome Biol, 2016. 17: p. 72. (PubMed PMID: 27091476)
28. Kim C, et al. Chemoresistance Evolution in Triple-Negative Breast Cancer Delineated by Single-Cell Sequencing. Cell, 2018. 173(4): p. 879-93 e13. (PubMed PMID: 29681456)
29. Clark SJ, et al. scNMT-seq enables joint profiling of chromatin accessibility DNA methylation and transcription in single cells. Nat Commun, 2018. 9(1): p. 781. (PubMed PMID: 29472610)
30. Guo F, et al. Single-cell multi-omics sequencing of mouse early embryos and embryonic stem cells. Cell Res, 2017. 27(8): p. 967-88. (PubMed PMID: 28621329)